CSDMS history
A Brief History of CSDMS
Introduction
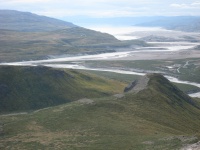
The CSDMS Project is an NSF-funded, international effort to develop a suite of modular numerical models able to simulate the evolution of landscapes and sedimentary basins, on time scales ranging from individual events to many millions of years. CSDMS is an acronym for Community Surface Dynamics Modeling System. Ideas behind the CSDMS concept were discussed by participants of an international workshop, Numerical Experiments in Stratigraphy (University of Kansas, May 15-17, 1996), with formal presentation of these findings at the third annual conference of the International Association of Mathematical Geology (Barcelona, 1997: Syvitski, et al, 1997). The formal CSDMS idea, however, took shape at a panel convened by the Geology/Paleontology Program of NSF in March 1999. That panel identified a CSDMS as a high priority NSF research initiative in sedimentary geology, and since then the concept has been widely discussed in the North American sediment-dynamics community.
History
The history behind CSDMS began in the mid-1960s, with a very interesting article (Bonham-Carter and Sutherland, 1967; also see Harbaugh and Bonham-Carter, 1970). Graeme Bonham-Carter coded up sediment transport equations related to a river's discharge into the ocean, to provide us with new insights into the formation of sedimentary deposits. The exercise was completed at a time when application of the Navier-Stokes equation to sediment transport remained in its infancy, and when we fed computer cards into memory-poor, slow-speed mainframes. Ten years later saw the first volume describing the full spectrum of numerical models related to ocean dynamics (Goldberg et al, 1977). The emphasis of these articles was on getting the dynamics correct and this resulted in some papers (e.g. Smith, 1977; Komar, 1977) being conceptually ahead of available field tools and data.
Through the next decade, as computers advanced with our ability to develop code, the softrock community applied its maturing understanding of hydraulics and sediment transport to the formation and modification of sedimentary deposits. In 1988, a large representation of this community met in the mountains of Colorado, and the concept of quantitative dynamic stratigraphy (QDS) was born (Cross, 1989). At the meeting, a mechanistic view of QDS was contrasted with the more rapidly maturing rule-based (sometimes known as geometric-based) stratigraphic models (see Syvitski, 1989, for discussion). The need to understand local to regional boundary conditions, either over long periods of simulated time, or for conditions where we have little field data (i.e. extreme event modeling) went on to change the way sedimentologists conducted their field and numerical experiments.
Through the next decade, the QDS community and discipline grew and influenced the field of both sedimentology and stratigraphy (Agterberg and Bonham-Carter, 1989; Martinez and Harbaugh, 1993; Franseen et al., 1991; Harff et al., 1998; Harbaugh et al., 1999; Paola, 2000; Syvitski and Bahr, 2001, Syvitski et al.,, 2007). Now we approach a time when these marvelous individual efforts can be multiplied in their effectiveness if better coordinated, and openness is developed between the modelers and field-oriented geoscientists.
The earth system surface dynamic models, like the established Community Climate System Model or the Regional Ocean Modeling System, are based on algorithms that mathematically describe the processes and conditions relevant to water, sediment and solute transport, and would incorporate all the important input and boundary conditions that define an environmental system. The CSDMS effort is coordinated and funded by government agencies and industry, to support the development of optimum algorithms, input parameters, feedback loops, and observations at the relevant scales necessary, to better provide an understanding of earth-surface systems. The Community Surface Dynamics Modeling System is being designed to address issues, for example, when the earth was abiotic, hotter or colder, when there was no flocculation, when the moon was closer, or the oceans were more saline. CSDMS provides modeling support to those working on modern environmental applications, future global warming scenarios, natural disaster mitigation efforts, natural hazard efforts, reservoir characterization, oil exploration, and national security. New satellite and geophysical databases will only realize their full potential in collaboration with efforts like the Community Surface Dynamics Modeling System.
More About the CSDMS Project
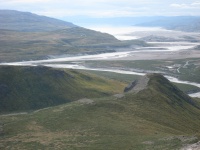
The world's media make frequent reference to "the environment", but rarely do we ask exactly what is meant by this term. Often what is meant is the Earth's surface, with its interwoven physical, biological, and chemical systems, all overprinted by human influences. The Earth's surface itself is the cradle and the arena for its biological systems, from terrestrial alpine regions to the depths of the ocean. The physical, chemical, and biological systems of the Earth's surface are so deeply interwoven that the surface is a kind of "living skin" of our planet.
Most of us tend to think of the surface as relatively static. But, viewed on an appropriate time scale, our planet's surface is dynamic in ways that parallel the more familiar dynamism of the atmosphere or the oceans. And the time scale need not be very long. Dramatic events like landslides can occur in seconds. Subtle but common forms of landscape change can control nutrient flow and population stability, especially in steep terrains. Over years to decades, changes in critical surface features like beaches and rivers can affect large areas and the populations that inhabit them. We need only extend our time horizon slightly to see that a quantitative understanding of surface dynamics is the cornerstone of environmental science.
The main goal of CSDMS is to offer a community-built and freely available suite of integrated, ever-improving software models and modules that predict the movement of fluids, and the flux (production, erosion, transport, and deposition) of sediment and solutes in landscapes and their sedimentary basins.
Another goal is to encourage more open collaboration and cooperation within this modeling community. Working together we hope to make the earth surface dynamics modeling community more effective with less duplication of effort, more ways to connect models, and open source models that allow others to easily build upon them. Not long ago the climate modeling community experienced such a cultural shift, which resulted in better funding and vastly improved, integrated modeling.
To be successful CSDMS must nurture, develop and maintain a high-level of community participation and enlist modelers who can benefit the entire earth surface modeling community. CSDMS will strive to do this via:
- Well-documented and user-friendly LBE software that demonstrably keeps pace with both hardware and scientific developments;
- Partnerships with related computational and scientific programs in order to 1) eliminate duplication of effort, and 2) leverage mutual progress (i.e., to provide and benefit from an intellectually stimulating environment);
- Appropriate training for both the users and teaching communities;
- Hardware and personnel resources to support and facilitate software development and its use by the community;
- A strong link between what is predicted by CSDMS codes and what is observed both in nature and in physical experiments;
CSDMS will develop and maintain the computational system, and it will ensure the portability and interoperability of modules, the computational efficiency of system code, and the clarity and consistency of documentation, interfaces, etc. CSDMS will also offer pedagogically evaluated LBE technology to enhance and inform education in environments of high school, undergraduate programs, and science museums.
Three proof-of-concept challenges are being addressed: 1) Predicting the transport and fate of fine sediment and carbon from source areas in the landscape to sedimentary basins that accumulate sediment over time; 2) Understanding the impact of humans on the natural cycle, through, for example, changes in waterways, forest fires, and agricultural practices; and 3) Tracking the evolution of landscapes and seascapes through the geological past, with focus on Pleistocene glacial cycles.
References
Syvitski, J., Pratson, L., Perlmutter, M., de Boer, P., Parker, G., Garcia, M., Wiberg, P., Steckler, M., Swift, D., and Lee, H.J., 1997. EarthWorks: A large scale and complex numerical model to understand the flux and deposition of sediment over various time scales. In: V. Pawlowsky-Glahn (Editor) Proceedings of IAMG’97 The third annual conference of the International Association of Mathematical Geology. CIMNE-Barcelona, 1997, v. 3 p. 29-33.
Bonham-Carter, G.F. and Sutherland, A.J., 1967. Diffusion and settling of sediments at river mouths: A computer simulation model: Transactions of the Gulf Coast Association of Geological Societies, v. 17, p. 326-338.
Harbaugh, J.W., Whatney, L.W., Rankay, E., Slingerland, R., Goldstein, R., and Franseen, E. editors, 1999. Numerical Experiments in Stratigraphy: Recent Advances in Stratigraphic and Computer Simulations. SEPM special publication. No. 62, 362 p.
Goldberg, E.D., McCave, I.N., O’Brien, J.J. and Steele, J.H. editors, 1977. The Sea, Volume 6, Marine Modeling. New York, Wiley Interscience.
Smith, J.D., 1977, Modeling of sediment transport on continental shelves, in Goldberg, E.D., McCave, I.N., O'Brien, J.J., and Steele, J.H., eds., The Sea Volume 6, Marine Modeling: Wiley Interscience, New York, p.539 - 577.
Komar, P.D., 1977, Computer simulation of turbidity current flow and the study of deep -sea channels and fan sedimentation, in Goldberg, E.D., McCave, I.N., O'Brien, J.J., and Steele, J.H., eds., The Sea Volume 6, Marine Modeling: Wiley Interscience, New York, p. 603 - 621.
Cross, T.A. editor, 1989. Quantitative Dynamic Stratigraphy, Englewoods Cliff, NJ, Prentice Hall, 624 p.
Syvitski, J.P.M., 1989. The process-response model in Quantitative Dynamic Stratigraphy. In: T.A. Cross (ed.) Quantitative Dynamic Stratigraphy. Prentice-Hall, N.Y., p. 309-334.
Agterberg, F.P. and Bonham-Carter, G.F., editors, 1989. Statistical Applications in the Earth Sciences. Geological Survey of Canada Paper 89-9, 588 p.
Martinez, P.A. and Harbaugh, J.W., 1993. Simulating Nearshore Environments. Computer Methods in Geosciences v. 12, Pergamon Press, 265 p
Franseen, E.K., Watney, L.W., Kendall, C.G., and Ross, W., editors, 1991. Sedimentary Modeling: Computer simulations and methods for improved parameter definition. Kansas Geological Survey, Bulletin 233, 524 p.
Harff, J., Lemke, W., and Stattegger, K., editors, 1998. Computerized Modeling of Sedimentary Systems. Springer-Verlag, Berlin, 452 p.
Harbaugh, J.W., Whatney, L.W., Rankay, E., Slingerland, R., Goldstein, R., and Franseen, E. editors, 1999. Numerical Experiments in Stratigraphy: Recent Advances in Stratigraphic and Computer Simulations. SEPM special publication. No. 62, 362 p.
Paola, C., 2000, Quantitative models of sedimentary basin filling: Sedimentology, v. 47 (suppl. 1), p. 121-178.
Syvitski, J.P.M., and Bahr, D.B., editors, 2001. Numerical Models of Marine Sediment Transport and Deposition. Computers and Geoscience Special Issue. 27(6): 617-753.
Syvitski, J.P.M., Pratson, L.F., Wiberg, P.L., Steckler, M.S., Garcia, M.H., Geyer, W.R., Harris, C.K., Hutton, E.W.H., Imran, J., Lee, H.J., Morehead, M.D., and Parker, G., 2007. Prediction of margin stratigraphy. In: C.A. Nittrouer, J.A. Austin, M.E. Field, J.H. Kravitz, J.P.M. Syvitski, and P.L. Wiberg (Eds.) Continental-Margin Sedimentation: From Sediment Transport to Sequence Stratigraphy. IAS Spec. Publ. No. 37: 459-530.