Form:Meetingconfirmation
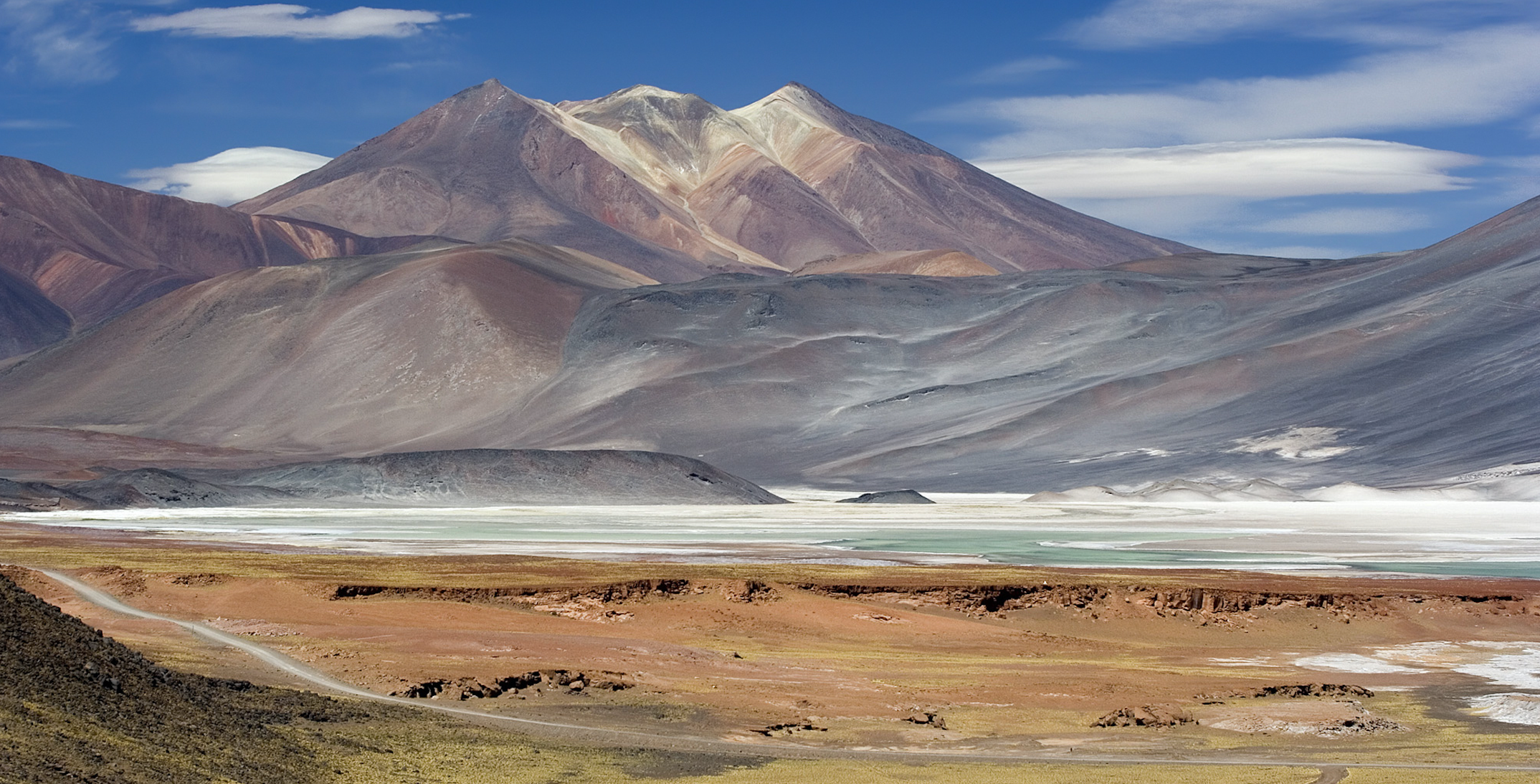
Confirmation
Confirming your participation in the workshop is now closed. Please contact Lynn McCready: csdms@colorado.edu with subject title: "CTSP Meeting 2018" if you have any questions.
Introduction
A workshop focused on Coupling of Tectonic and Surface Processes (CTSP) will be held from April 25-27, 2018 and is intended to survey both questions and state of the art numerical techniques that simulate surface processes and long term tectonic (LTT) processes in an attempt to define a framework for the development of efficient numerical algorithms that couple across multiple length and time scales. This workshop will provide a unique opportunity for researchers to develop collaborations and proposal ideas and by doing so enhance and increase the impact of both the CIG and CSDMS communities. We expect a broad and diverse audience drawn from domestic and international research communities, including graduate students, post-docs, and early career scientists, who are interested in coupling landscape evolution to tectonic processes.
The workshop will occur over 2 ½ days at the University of Colorado (Boulder) and will soon be open for applications. The first two days will be dedicated to a survey of existing questions and numerical techniques and challenges through a combination of breakout discussions and presentations by leading experts in the field. The last ½ day will be dedicated to developing a white paper that outlines different mechanisms through which the LTT and surface processes communities can collaborate to tackle the science questions and the numerical challenges defined over the first two days. The hope is that such a white paper will serve to set the stage for new educational and method development efforts, including the submission of a NSF Research Collaboration Network proposal.
Agenda
Click here to view the final agenda of 04/19/2018.
Remote Participation
Registration is required in advance (there is no fee for remote participants). Once you have registered, a link will be forwarded with detailed remote joining instructions.
Please complete the registration process for each session you would like to attend:
Wednesday, April 25th
Morning Session 8:30AM to 12:00PM (MDT): Registration URL: https://cuboulder.zoom.us/meeting/register/2ec271668d7b1fd57c24e00bf0acd2b8
Afternoon Session 1:00PM to 4:15PM (MDT) Registration URL: https://cuboulder.zoom.us/meeting/register/b5b5cd49b2e1ea67d746f627e8486654
Thursday, April 26th
Morning Session 8:30AM to 12:00PM (MDT) Registration URL: https://cuboulder.zoom.us/meeting/register/bf4d266a7a79d67666858a512be5123a
Afternoon Session 1:00PM to 5:00PM (MDT) Registration URL: https://cuboulder.zoom.us/meeting/register/3b2a0ef470874c17dc2040ba88984b7b
Keynote Speakers
Session 1: Big Questions
Alison Duvall
University of WashingtonFrom Earthquakes to Landscapes in the Cascadia Subduction Zone Increased computing power, high resolution imagery, new geologic dating techniques, and a more sophisticated comprehension of the geodynamic and geomorphic processes that shape our planet place us on the precipice of major breakthroughs in understanding links among tectonics and surface processes. In this talk, I will use University of Washington’s “M9 project” to highlight research progress and challenges in coupled tectonics and surface processes studies over both short (earthquake) and long (mountain range) timescales. A Cascadia earthquake of magnitude 9 (M9) would cause shaking, liquefaction, landslides and tsunamis from British Columbia to northern California. The M9 project explores this risk, resilience and the mechanics of Cascadia subduction. At the heart of the project are synthetic ground motions generated from 3D finite difference simulations for 50 earthquake scenarios including factors not previously considered, such as the distribution and timing of energy release on the fault, the coherent variation of frequency content of fault motion with fault depth, and the 3D effects of the deep basins along Puget Sound. Coseismic landslides, likely to number in the thousands, represent one of the greatest risks to the millions of people living in Cascadia. Utilizing the synthetic ground motions and a Newmark sliding block analysis, we compute the landscape response for different landslide failure modes. Because an M9 subduction earthquake is well known to have occurred just over 300 years ago, evidence of coseismic landslides triggered by this event should still be present in Washington and Oregon landscapes. We are systematically hunting for these landslides using a combination of radiocarbon dating and surface roughness analysis, a method first developed to study landslides near to the Oso 2014 disaster site, to develop more robust regional landslide chronologies to compare to model estimations. Resolved ground motions and hillslope response for a single earthquake can then be integrated into coupled landscape evolution and geodynamic models to consider the topographic and surface processes response to subduction over millions of years. This example demonstrates the power of an integrative, multidisciplinary approach to provide deeper insight into coupled tectonic and surface processes phenomena over a range of timescales. Katherine Barnhart
University of Colorado, BoulderTesting landscape evolution models with natural and synthetic experiments From G.K. Gilbert's "The Convexity of Hilltops" to highly-optimized numerical implementations of drainage basin evolution, models of landscape evolution have been used to develop insight into the development of specific field areas, create testable predictions of landform development, demonstrate the consequences of our current theories for geomorphic processes, and spark imagination through hypothetical scenarios. In this talk, I discuss how the types questions tackled with landscape evolution models have changed as observational data (e.g., high-resolution topography) and computational technology (e.g., accessible high performance computing) have become available. I draw on a natural experiment in postglacial drainage basin incision and a synthetic experiment in a simple tectonic setting to demonstrate how landscape evolution models can be used to identify how much information the topography or other observable quantities provide in inferring process representation and tectonic history. In the natural example, comparison of multiple calibrated models provides insight into which process representations improve our ability to capture the geomorphic history of a site. Projections into the future characterize where in the landscape uncertainty in the model structure dominates over other sources of uncertainty. In the synthetic case, I explore the ability of a numerical inversion to recover geomorphic-process relevant (e.g., detachment vs. transport limited fluvial incision) and tectonically relevant (e.g., date of fault motion onset) system parameters.
Session 2: Time & Length Scales
Jean-Arthur Olive
ENS ParisFeedbacks between brittle deformation and surface processes: Insights from extensional settings Major fault systems are the primary manifestation of localized strain at tectonic plate boundaries. Slip on faults creates topography that is constantly reworked by erosion and sediment deposition. This in turn affects the stress state of the brittle upper crust. Numerical models commonly predict that surface processes can modulate the degree of strain localization, i.e., the partitioning of strain onto a given number of master faults and/or the lifespan of individual faults. The detailed mechanisms, potential magnitude, and geological evidence for such feedbacks however remain debated. We address this problem from the perspective of continental rifts, and at the scale of individual fault-bounded structures. Half-grabens in particular constitute ideal natural laboratories to investigate brittle deformation mechanisms (e.g., fault localization, elasto-plastic flexure...) in relation to continued erosion of the master fault footwall and sediment deposition on the hanging wall. Through an energy balance approach, we show that suppressing relief development in a half-graben can significantly enhance the lifespan of its master fault if the upper crust is moderately strong. Simple geodynamic simulations where tectonic topography is either entirely leveled or perfectly preserved confirm our analytical predictions.
Natural systems, however, lie somewhere in between these two endmembers. To better represent the true efficiency of surface processes at redistributing surficial masses, we couple a 2-D long-term tectonic code with a landscape evolution model that incorporates stream power erosion, hillslope diffusion, and sediment deposition. We identify a plausible range of landscape evolution parameters through morphological analyses of real normal fault-bounded massifs from the East African Rift and Western United States. This allows us to assess the sensitivity of half-graben evolution to a documented range of rheological, climatic, and lithological conditions. We find that half-grabens that reach topographic steady-state after a short amount of extension (~1 km) are more likely to accumulate master fault offsets on par with the thickness of the upper crust. Conversely, a longer phase of topographic growth ––for example due to low rock erodibility–– will favor the initiation of a new master fault and the abandonment of the initial one. A less erodible crust could thus be more prone to extension on a series of horsts and grabens, while more erodible units would deform as long-lived half-grabens. Lithological controls on erodibility could therefore constitute a form of structural inheritance in all geodynamic contexts.Phaedra Upton
GNS ScienceCoupling geodynamics and surface processes The landscape serves as a nexus between the solid Earth with its geodynamic processes and the atmosphere. At many spatial and temporal scales landscape morphology and topography provide a constraint on the tectonics of the deeper Earth and the processes active or previously active within it. In order to unravel these, we need to understand the complex relationships between surface processes, their drivers and the Earth materials on which they act.
In my talk, I will explore recent developments in modelling surface processes within a single deformational framework. I will focus on collisional settings such as New Zealand’s Southern Alps, SE Alaska and the Himalaya where rapid uplift combines with vigorous climate regimes to create dynamic landscapes. Topics will include:- Exploring the complete stress tensor (tectonic, dynamic, topography, fluvial, glacial)
- Rock strength controls on topography and erosion rates
- Failure Earth Response Model
- Smooth particle hydrodynamics and its application to landscape evolution modelling
Jean Braun1,2, Xiaoping Yuan1, Audrey Margirier1, Eric Deal3, Kim Huppert1, Igor Lisac1, Frederic Herman4, Günther Prasicek4, Katerhine Kravitz1 & Jiao Ruohong1
.Parameterizing Surface Processes and their Response to Tectonic and Climatic Forcings 1Helmholtz Centre Potsdam, GFZ German Research Center for Geosciences, Potsdam, Germany
2Institute of Earth and Environmental Science, University of Potsdam, Potsdam, Germany
3Department of Earth, Atmospheric and Planetary Sciences, Massachusetts Institute of Technology, Cambridge, MA, USA
4Institute of Earth Surface Dynamics, University of Lausanne, Lausanne, Switzerland
The Earth’s topography is the product of surface vertical motions caused by tectonic processes and modulated by erosional processes that cause redistribution of mass at the Earth’s surface over a wide range of scales. The efficiency of these gravity-driven processes scales with slope (and thus topography). This also implies that the time scale needed for an orogenic system to reach steady-state between tectonic uplift and erosion must scale with the height of the topography, the tectonic uplift rate and the degree of isostatic compensation. Using simple observations from a range of presently active orogenic systems, we estimate that this time scale is comprised between 1 and 15 Myrs. From these estimates, we can also derive a “generic” erosion law based on the Stream Power Law (SPL) to be used in geodynamic models. We also show that the degree of non-linearity of the erosional laws (i.e., the value of the slope exponent) controls the rate at which topography decays once tectonic uplift ceases. This simple behavior of any slope-dependent erosion law may explain the post-orogenic longevity of Earth’s topography.
The same processes that shape the Earth’s topography are, for the most, functions of the availability of moisture from the atmosphere. This has led to the conclusion that there must be a strong link between the efficiency of surface processes and climate. This link is however difficult to establish from observational evidence. For example, the effect of the Cenozoic cooling of the Earth’s climate on the efficiency of erosion in orogenic systems remains highly debated. We propose that this question is difficult to address because the wide range of erosional processes active at the Earth’s surface (such as fluvial erosion, hillslope processes, glacial abrasion, peri-glacial processes, chemical weathering, etc.) are characterized by different response times to climate perturbations. These response times may also depend on the dimensions of the topographic feature being eroded, the mean slope, the mean precipitation (or accumulation) rate and the nature of the rocks being eroded. It is therefore not surprising that a global correlation between climate change and erosional efficiency is difficult to evidence.
Recent work has also shown that erosional efficiency is strongly dependent on the variability of climate and, in particular, of precipitation. We will show how this climate variability has been introduced in fluvial erosional models using a simple stochastic approach. This requires, however, that mean precipitation and precipitation variability (or storminess) be translated into mean discharge and discharge variability. This can be achieved through the use of an eco-hydrological model that requires a limited number of parameters only.
To conclude we will use a surface processes model to demonstrate how tectonics, surface processes and climate interact with each other over geological time scales to create landforms that will ultimately exert a strong control on biodiversity, species richness and endemism. We will illustrate this point using the island of Madagascar as a case example.
Session 3: Implementation science challenges
Brian Yanites
Indiana University-BloomingtonReconciling landscape models with reality: a spectrum of success The development of quantitative models of landscape evolution requires a rigorous assessment of how well they represent natural system dynamics. For some locations and processes, the models have been shown to provide reasonable representations of landscape dynamics over a range of timescales; however, in a number of cases, rather straightforward tests suggest our simplified approaches may require further development. I discuss some of these lingering issues in quantitative geomorphology with a focus on the ubiquitous ‘stream power model’ and show how different approaches offer potential solutions to implementation in landscape evolution models. I will show examples focusing on how climate and lithology are represented in landscape evolution models as well as discuss the limitations of representing a river channel with a 1-dimensional model. I summarize by suggesting that an iterative approach of numerical predictions, rigorous field assessment, and adjustment of theory is needed to fully capture and understand the dynamics of landscapes. Louis Moresi, Ben Mather, Romain Beucher
Melbourne UniversityQuagmire - Algorithms and a Toolbox for Parallel Surface Process Codes Background
When it comes to building a general, efficient, surface process code, there are a couple of significant challenges that stand in our way. One is to address the interesting operators that appear in the mathematical formulation that are not commonly encountered in computational mechanics. The other is to cater for the many different formulations that have been put forward in the literature as no single, universal set of equations has been agreed upon by the community.
Computational Approach
We view Quagmire as a community toolbox and acknowledge that this means there is no one best way to formulate any of the landscape evolution models. We instead provide a collection of useful operators and examples of how to assemble various kinds of models. We do assume that:- the surface is a single-valued height field described by the coordinates on a two-dimensional plane
- the vertical evolution can be described by the time-derivative of the height field
- the horizontal evolution can be described by an externally imposed velocity field
- the formulation can be expressed through (non-linear) operators on the two dimensional plane
- any sub-grid parameterisation (e.g. of stream bed geometry) is expressible at the grid scale
- a parallel algorithm is desirable
- the nature of the mesh: triangulation or a regular array of 'pixels'
- the parallel decomposition (except that it is assumed to be general)
- the specific erosion / deposition / transport model
Mathematical Approach
Matrix-vector multiplication is the duct tape of computational science: fast, versatile, ubiquitous. Any problem that can be formulated in terms of basic linear algebra operations can usually be rendered into an abstract form that opens up multiple avenues to solve the resulting matrix equations and it is often possible to make extensive use of existing fast, parallel software libraries. Quagmire provides parallel, matrix-based operators on regular Cartesian grid and unstructured triangulations for local operations such as gradient evaluation, diffusion, smoothing but also for non-local, graph-based operations that include catchment identification, upstream summation, and flood-filling.
The advantage of the formulation is in the degree of abstraction that is introduced, separating the details of the discrete implementation from the solution phase. The matrix-based approach also makes it trivial to introduce concepts such as multiple-pathways in graph traversal / summation operations without altering the structure of the solution phase in any way. Although we have not yet done so, there are obvious future possibilities in developing implicit, non-linear solvers to further improve computational performance and to place the model equations in an inverse modelling framework.
Session 4: Numerical techniques/Computational Science Challenges
Mark Piper
Community Surface Dynamics Modeling System (CSDMS), University of Colorado BoulderCommunity cyberinfrastructure for modelling Earth-Surface processes Understanding the processes that shape and reshape the earth's surface is a fundamental challenge in the geosciences. Numerical modeling—the glue between data and theory—is a key component of the effort to meet this challenge. The Community Surface Dynamics Modeling System (CSDMS) was formed to provide support for earth-surface dynamics modeling, and to accelerate the pace of discovery through software development, resource sharing, community coordination, knowledge exchange, and technical training.
The CSDMS approach is bottom-up: models, typically developed within the community, are nominated by the community for inclusion within the CSDMS Modeling Framework (CMF). The CMF provides loose, two-way coupling in a Python-based framework that can scale from an individual laptop to a high-performance computing environment. The CMF has a web-based front-end, the Web Modeling Tool (WMT), that's available for use by all community members.
The CMF is built on four key software technologies:- Basic Model Interface. A Basic Model Interface (BMI), consisting of a common set of functions for initializing, running, and finalizing a model, is added to each model to be incorporated into the CMF.
- Standard Names. Given variables from two models, Standard Names provides a semantic matching mechanism for determining whether—and the degree to which—the variables refer to the same quantity.
- Babel. C, C++, Fortran, Java, and Python language bindings for a BMI-enabled model are generated by Babel.
- Python Modeling Toolkit. The Python Modeling Toolkit (PyMT) is the framework part of the CMF, allowing Babel-wrapped models to be coupled and run in a Python environment. PyMT includes tools for time interpolation, grid mapping, data exchange, and visualization.
In this presentation, I'll provide an overview of these core CSDMS software technologies, describing the problems they solve, how they benefit the community, and how they may accelerate scientific productivity. I'll include a Jupyter Notebook demonstration of using PyMT to interactively couple and run a landscape evolution model with a sediment transport model. I'll conclude with a list of issues still to be addressed by CSDMS.John Naliboff, Juliane Dannberg, Rene Gassmoeller, Lorraine Hwang, Louise Kellogg
Computational Infrastructure in Geodynamics (CIG), University of California, DavisMethods, challenges and uncertainty in modeling tectonic processes The computational geodynamics community uses a diverse suite of methods and software to model solid Earth deformation across a wide range of spatial and temporal scales. Within the discipline of computational tectonics, a wide variety of options exists to model non-linear deformation of the lithosphere and convecting mantle, which range from CIG-supported software to widely used software by individual research groups to closed-source proprietary commercial codes. This presentation will review the available methods, open-source community resources, state-of-the-art applications and some of the numerical challenges associated with modeling non-linear tectonic processes. In particular, we will highlight the wide range of difficulties for efficiency, accuracy, computational analysis, storage, reproducibility, uncertainty quantification and verification. Last, we will present some solutions to these challenges in the context of multi-physics simulations and code coupling, with an outlook towards integration between the surface processes and lithospheric dynamics community.
Participants
- As of 05/17/2024, 93 participants registered for the workshop. Click here to view the participant list.
- Participants abstracts can be downloaded here.
Organizing committee
Mark Behn | Luc Lavier |
Woods Hole Oceanographic Institution (WHOI) | University of Texas - Austin |
Thorsten Becker | Phaedra Upton |
University of Texas - Austin | GNS - New Zealand |
Eric Mittelstaedt | Catherine Cooper |
University of Idaho | Washington State University |
Greg Tucker | Louise Kellogg |
University of Colorado - Boulder | University of California - Davis |
Nicole Gasparini | Boris Kaus |
Tulane University | Johannes Gutenberg University - Mainz |
Lorraine Hwang | |
University of California, Davis |
Funding
This workshop is funded by the National Science Foundation through the Earth Sciences Division. There is no registration fee. We will be able to cover most on-site expenses (venue costs, hotel expenses based on double occupancy, and breakfast & lunch) for approximately 70 participants. Most participants will have their travel to and from the meeting covered. We will not be able to provide dinners on-site, but there are several restaurants nearby. Confirmed participants whose on-site expenses are covered are expected to arrive on Tuesday evening and leave on Friday morning.
Important dates
- Late November: Applications are opened
- January 15th: Application deadline
- February 1st: Successful applicants are invited to confirm participation
- February 15th: Deadline for confirmation of attendance
- Late March: Final meeting agenda is released